Tesla, Edison and the War of Currents
By Michael Parks, PE, for Mouser Electronics
The latter part of the 19th century saw the first footsteps in a technological revolution that would dwarf all the advances made up to that point. Electrification profoundly changed every aspect of human life. Not only did electrification change existing technologies of the time (oil lighting to electric light bulbs), but it also created entirely new products previously undreamt of such as radio. While we take our current electricity-based infrastructure and society for granted today, the path to this point was not as straightforward as we might like to remember. In the late 1880’s there was a “format war” raging much like our modern day VHS versus Betamax and Blu-Ray vs HD-DVD skirmishes. In those years Alternating Current (AC), whose figurehead was the Serbian-American eccentric genius Nikola Tesla, was battling Direct Current (DC) and it’s brilliant but arguably more business savvy Thomas Edison. In the end compromises were struck and electrification of the world was able to jump from blueprint to reality.
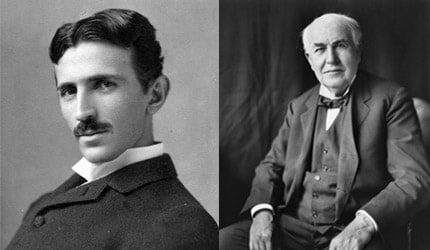
Figure 1: Nikola Tesla (left) and Thomas Edison (right)
AC vs DC
At the heart of the argument, the utility of AC versus DC comes down to good old engineering tradeoffs. In the 1880’s DC was less efficient to transport over long distances since line losses were significant compared to the DC voltage levels that could be generated. Thus powering the world with DC would require a larger amount of power generating stations dotting the landscape. However, for 1880’s machinery generating direct current was much simpler, giving it somewhat of a technological advantage. In contrast, alternating current required much more precise machinery to generate the oscillating electrons but AC transmission was far more efficient over large distances. These two solutions very much reflect the mindsets of their champions. Tesla tended to be more theoretical and able to invent in his mind’s eye whereas Edison was the consummate hands-on maker of his day. Elegant yet delicate design versus utilitarian practicality. In the end when it came to pushing electrons from power plant to consumer, alternating current seized victory from direct current.
AC Victory for Transmission
The key advantage that alternating current has is that the voltage level can be raised very easily with relatively inexpensive transformers. Higher voltage allows the same amount of power to be transmitted with less current. This translates to very practical advantages. With a smaller line loss, the distance between the consumer and the power generating facility can be far greater. Having to build out a smaller infrastructure meant that it was more cost-effective and practical to use AC for power transmission.
Of course, Edison would not go down without a fight. In the latter part of the 19th century Edison famously held demonstrations in which AC was used to electrocute animals in an attempt to persuade the masses of the dangers of alternating current. In addition, the first electric chair was built for New York State by two Edison employees. Unsurprisingly, alternating current was chosen to power the chair. While these demonstrations made for good showmanship, the reality is that both DC and AC can be dangerous if the current is sufficiently large. It only takes a few milliamperes of current in the right conditions to cause heart fibrillations that lead to death.
Over the years, refinements in technology have led to an improved performance in our electric grid. Electromagnetic Interference (EMI) caused by line noise upstream and loads connected downstream can “dirty” the power. Filters based on common mode chokes and capacitors from companies such as EPCOS/TDK help to reduce EMI which is critical as electronics devices increasingly require cleaner power to operate.
Figure 2: Chokes help to keep power lines clear of EMI. Image courtesy of TDK.
DC and the Digital Revolution
While alternating current may have become the de facto standard for power transmission and distribution, direct current has maintained many crucial roles in modern life. DC motors dominate many low horsepower mechanical applications. Direct current also plays a crucial role in telecommunications devices and the automobile systems (battery and inverter to convert the alternators AC to DC).
Perhaps the biggest victory for DC coincided with the digital revolution that began in earnest in the 1970’s after decades in laboratories around the world. Digital technology is absolutely dependent on DC power. The 1’s and 0’s that power the information age rely on the presence or absence of a direct current voltage. For digital devices that get their power from an AC wall outlet, the alternating current must first be transformed in direct current. Rectifier circuits are used to perform this function in modern day devices.
For mobile digital technology that has cut the wires, batteries provide the direct current needed. Batteries rely on electrochemical reactions of its constituent components to generate a constant stream of electrons at a given voltage level. Battery life is a function of the mA-H rating of the battery and the current draw of the device. Switched DC-DC converters used in modern day mobile electronics allow for the fixed DC voltage level of battery to service multiple sub-circuits that may operate at different voltages levels. One negative side effect of switched mode DC-DC converters is the creation of RF noise that must be suppressed using special components such as RF chokes. Today, aside from our larger appliances the vast majority of the devices we bring into our home ultimately rely on direct current to perform their function.
The Future
Much has changed in the almost 150 years in terms of quality and efficiency of the equipment that powers modern life. However, the fundamental concepts have remained by and large the same. Large power generation facilities rely on converting various energy sources into electricity which is then transmitted along an electrical grid. As the electricity nears the consumer is stepped down to lower and lower voltages until it is fed into your building through a final step-down transformer and circuit breaker panel, lastly arriving at the AC wall outlet. Our devices either consume the AC directly or transform it into a more useful DC voltage that brings our electronics to life.
Figure 3: Tesla's Wardenclyffe Tower (Image courtesy of Wikipedia)
One methodology that has garnered much attention (and perhaps even a cult-like following) is wireless power transfer. To clarify what is meant by wireless transfer we aren’t talking products like inductive smartphone chargers. These devices do indeed wirelessly transmit power, though over very short distances and are driving relatively low power devices. Rather, the notion in question is whether or not the world’s entire electrical grid could be replaced with antennas that radiate electrical power much like one can “scoop” radio and broadcast television signals from the air. The altruistic-minded Nikola Tesla himself was enamored with just such a concept going so far as building the Wardenclyffe Tower on Long Island to experiment with the ideas of transmitting both information (voice and facsimiles) and power. The fundamental problem with wireless power transmission is the math. The inverse-square law for an isotropic radiator (an antenna that radiates energy equally in all three dimensions) tells us the following:

According to the International Energy Agency, in 2012 the world consumed 20,900 TWh of electricity. Using a very rudimentary calculation based on the inverse-square formula, it would be necessary to generate 5.1x1014 times more energy than the 20,900 TWh to account for the transmission losses. In other words, the economics and physics demonstrate that wireless power transfer is completely impractical.
So if wireless power transmission to every home, school, store and factory remains a pipedream; what are the changes that might actually come to change our world? To look forward, sometime we have to look backwards.
At the same time as Edison and Tesla (and his champion George Westinghouse) were fighting the AC versus DC battles, other concepts were being explored. High Voltage DC (HVDC) technologies were being explored as early as the 1880s but the technology of the time meant HVDC equipment was expensive to manufacture and maintain. Flash forward a century later and technology began to catch up with the theory. The introduction of solid state technology (e.g. insulated-gate bipolar transistors and integrated gate-commutate thyristors from companies such as Infineon Technologies beginning in the 1970s meant conversion of AC to high voltage DC (as high as 800KV) became practical. While the AC-to-DC conversion technology at either end of an HVDC transmission system is expensive, the cost of the DC transmission hardware in between becomes much more economical at distances over 400 miles as you do not need to run cabling for three phases or account for skin effect. An HVDC system could also be used to interconnect unsynchronized AC transmission networks, adding some capacity handling capability lacking in current disconnected grids. However, system reliability for HVDC systems is not quite yet on par on with their AC counterparts. Coupled with the fact that HVDC technology is still advancing quickly, operating an HVDC network requires maintaining a significant spares inventory that may or may not be interoperable with various HVDC systems.
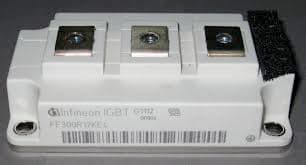
Figure 4: Solid state devices such as IGBTs from Infineon help to make HVDC a practical reality (Image courtesy of Infineon)
It’s also possible that the future of power transmission is no transmission hardware at all. Renewable energy sources are being increasingly harvested closer and closer to the energy consumer. It is no surprise that photovoltaic panels are popping up on the roofs of houses all across the world. As efficiency increases and breakthroughs in energy storage emerge, it is not unfathomable that living “off the grid” will become more common than not. But here is where it gets very interesting. Photovoltaics produce DC voltage. Therefore, to tie it into your home it must first be converted to AC. Significant losses are incurred at each conversion from AC to DC and vice versa. There is work being done through industry alliance, such as the EM Alliance to advocate for local DC distribution. In short, the wall outlet of tomorrow might just be a USB port and not the three prong AC outlet that is the standard today. In the end, it’s possible that Tesla won the most battles, but Edison will win the war.
The War That Never Was, Perhaps
The world of today has been undoubtedly shaped by the pioneering work of both Nikola Tesla and Thomas Edison. Modern life was made possible thanks to our ability to harness the power of the electron. Both AC and DC have found their respective use cases in our high tech world. We have learned how to exploit the technological and economic advantages and disadvantages of both technologies to our benefit. Fortunately for engineers and technology companies we are on the threshold of a second electrical revolution. As the world begins to seriously look at harnessing renewable sources to become better environmental stewards and energy independent, we will have to integrate solar, wind, geothermal, and tidal-based electricity generating technology into the grid. Understanding the advantages and disadvantages of AC and DC transmission with respect to how these sources generate electrons will be a key factor to our future success. It would seem both Tesla and Edison would be justly proud of the contributions that AC and DC offer to building a better tomorrow.
Michael Parks, P.E. is the owner of Green Shoe Garage, a custom electronics design studio and technology consultancy located in Southern Maryland. He produces the S.T.E.A.M. Power Podcast to help raise public awareness of technical and scientific matters. Michael is also a licensed Professional Engineer in the state of Maryland and holds a Master’s degree in systems engineering from Johns Hopkins University.